Why Measure Dissolved Oxygen?
DO is one of the most commonly measured water quality parameters, but the reason for measuring it varies based on the environment.
Why Measure Dissolved Oxygen in Surface Water and Aquaculture?
Dissolved oxygen is a direct indicator of a water body's ability to support aquatic life – aquatic organisms need DO to survive!
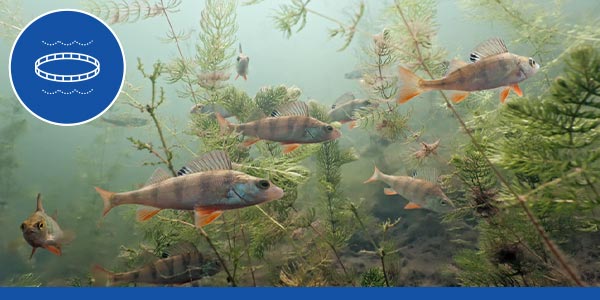
Figure 15: Fish require sufficient levels of dissolved oxygen to survive. Many species cannot survive if concentrations drop below 4 mg/L.
The level of DO required varies by species. In general, most fish species will grow and thrive within a range of 5-12 mg/L. However, if levels drop below 4 mg/L, they may stop feeding and become stressed, possibly leading to large fish kills. Hypoxia occurs when the concentration of dissolved oxygen decreases to a level that can no longer support living aquatic organisms.
Check out our blog post on Dissolved Oxygen Management and Related Costs in Pond Aquaculture to learn more about the importance of measuring DO in fish farming and other forms of aquaculture. We also created a Hypoxia Infographic that helps explain how hypoxia occurs in the environment.
An imbalance of DO occurs when there is a harmful algal bloom (HAB). During the early and peak growth phases of a HAB, DO can increase significantly in the vicinity of the bloom due to photosynthetic activity during the day. More oxygen is generated than can be consumed by either the algae or the other organisms, day or night – this can lead to supersaturation.
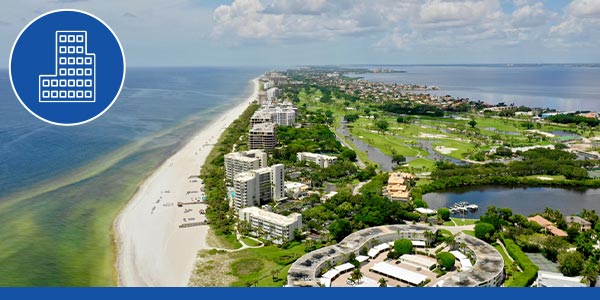
Figure 16: Harmful algae blooms (HABs) are often fed by nutrient runoff. Bacteria and other organisms consume the algae once the bloom dies, but this requires oxygen. DO levels can drop so low once the bloom dies that large fish kills can result..
As the bloom fades and dies, the algae become food for bacteria and other things that consume oxygen. This can cause DO levels to drop drastically, resulting in hypoxia. Check out our blog post, HABs | Everything You Need to Know, to learn more!
Large fish kills can also result from thermal pollution around power plants and industrial manufacturers. While the effluent from these plants is typically clean, it is often much, much warmer than the surface water it enters. As temperature increases, the level of DO in the water decreases. Therefore, a sudden influx of warm water can result in large fish kills.
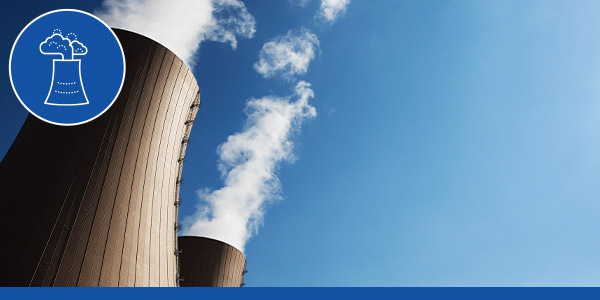
Figure 17: When hot water is released into a surface water body, dissolved oxygen levels drop. If the DO concentration decreases too much, fish kills can result. This is a big concern around power plants and industrial facilities.
Thermal pollution and HABs aren't the only events that endanger aquatic organisms. Road salt is commonly applied to icy roads in winter. This salt runs off the road and into surface water bodies, increasing salinity. As salinity increases, DO levels decrease. So, even though oxygen is more soluble in cold water, high salinity can result in large fish kills in winter due to suffocation.
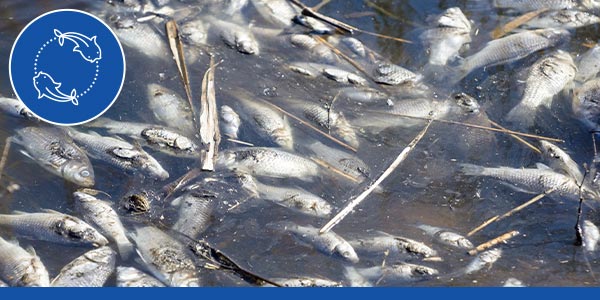
Figure 18: Road salt dissolved in a surface water body can wreak havoc on aquatic organisms, as the salt causes dissolved oxygen concentrations to decrease.
Why Measure Dissolved Oxygen in Groundwater?
Many assume DO is absent below the water table, but this is an incorrect assumption. Before water percolates downward from the surface, water is in contact with the atmosphere, and oxygen becomes dissolved. DO can exist at great depths in an aquifer as long as there is little or no oxidizable material.2
Dissolved oxygen can be a helpful parameter to measure when conducting groundwater investigations. DO can help determine when stable conditions have been reached during purging and can be used to evaluate well construction.
Measuring DO can also help ensure proper groundwater sampling procedures are being followed when collecting samples for the analysis of metal and volatile organic compounds. Any artificial aeration can impact laboratory analyses for these compounds.3
DO plays a significant role in chemical reactions that occur in the subsurface. It regulates the valence state of trace metals and constrains the metabolism of dissolved organic compounds (e.g., oil) by microbes.4
Microbes can degrade oil that has leaked into an aquifer. Like other organisms, microbes need to respire (i.e., breathe). Respiration requires an electron acceptor, and since oxygen is the most preferred one, DO is quickly depleted where there is contamination present. Therefore, DO can only be found outside a plume of contaminated groundwater.5
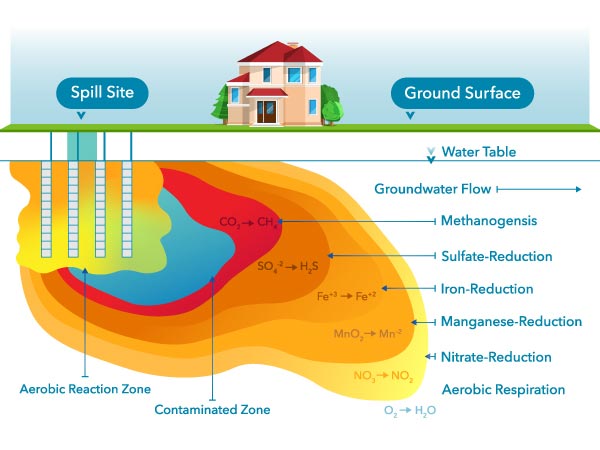
Figure 19: Dissolved oxygen is the preferred electron acceptor used by microbes during biodegradation of organic contamination in the subsurface. Once it is depleted, other electron acceptors are used by anaerobic microbes.5
Other electron acceptors are used once dissolved oxygen has been depleted. After oxygen, nitrate will be used up, so nitrate can only be found relatively far away from the plume, just like DO. The electron acceptor used last is carbon dioxide (CO2). The process of using CO2 is called methanogenesis; this will be occurring closest to the source of contamination.5
Other environments can become anoxic due to microbial activity, such as the open water contaminated by the Deepwater Horizon oil spill in 2010.
Why Measure Dissolved Oxygen in Wastewater?
Microbes consume waste and transform it into harmless end products in the treatment process at wastewater treatment plants. DO plays a critical role in this process, as these microbes rely on it to break down wastewater contaminants, such as organics or ammonia. In the activated sludge process (ASP) – the most common plant configuration – air is pumped into aeration tanks filled with microbes suspended in water.
Our blog post Wastewater or Water Resource Recovery? | Getting the Waste Out of Wastewater discusses aeration technology in more detail.
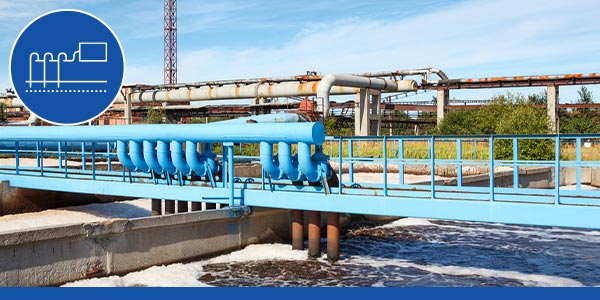
Figure 20: Air is pumped into aeration tanks to promote the degradation of wastewater contaminants by microbes.
Effluent, which is the treated water leaving the plant, must contain a limited amount of nutrients to ensure eutrophication does not occur in the environment. Biological nutrient removal (BNR) processes can be used to ensure compliance with nutrient effluent limits, but these processes require controlled conditions within the treatment plant.
BNR is characterized by the presence of unaerated anaerobic and anoxic zones upstream and downstream of aeration zones. Mixed liquor recycle and sludge return streams are arranged to make the best use of the organic content in the activated sludge system.
Check out our webinar on the Biological Nutrient Removal of Nitrogen to learn more about this treatment strategy.